Marie and Pierre Curie: We pick up the story in Marie and Pierre were married on July 26, and two years later she had her first child, a girl named Irene. Marie established that radioactivity was an atomic property, and was able to use it as a tool to search for new elements. The Curies managed to identify a distinct and previously unknown radioactive element within the pitchblende.
- Romanzo criminale (Einaudi. Stile libero big Vol. 1024) (Italian Edition)!
- Classroom Management: 24 Strategies Every Teacher Needs to Know.
- Radioactivity.
- Derrida, Badiou and the Formal Imperative (Continuum Studies in Continental Philosophy)!
- OCEANS (Endangered Biomes);
- Affirmationen: Transformation des Ichs (German Edition).
- Improbabilities?
The discovery of a second unknown ingredient came in December Much was to come from this…. Marie, on the other hand, could be considered the mother of radioactivity, thanks to her fascination with the energy suspended in the atoms she was studying. After four years of steady labor, Marie and Pierre Curie managed to extract a tenth of a gram of radium chloride on March 28, Background Info radioactive monticello.
Passing alpha particles through a very thin glass window and trapping them in a discharge tube allowed researchers to study the emission spectrum of the captured particles, and ultimately proved that alpha particles are helium nuclei. Other experiments showed beta radiation, resulting from decay and cathode rays , were high-speed electrons.
Likewise, gamma radiation and X-rays were found to be high-energy electromagnetic radiation. The relationship between the types of decays also began to be examined: For example, gamma decay was almost always found to be associated with other types of decay, and occurred at about the same time, or afterwards.
Gamma decay as a separate phenomenon, with its own half-life now termed isomeric transition , was found in natural radioactivity to be a result of the gamma decay of excited metastable nuclear isomers , which were in turn created from other types of decay. Although alpha, beta, and gamma radiations were most commonly found, other types of emission were eventually discovered. Shortly after the discovery of the positron in cosmic ray products, it was realized that the same process that operates in classical beta decay can also produce positrons positron emission , along with neutrinos classical beta decay produces antineutrinos.
In a more common analogous process, called electron capture , some proton-rich nuclides were found to capture their own atomic electrons instead of emitting positrons, and subsequently these nuclides emit only a neutrino and a gamma ray from the excited nucleus and often also Auger electrons and characteristic X-rays , as a result of the re-ordering of electrons to fill the place of the missing captured electron. These types of decay involve the nuclear capture of electrons or emission of electrons or positrons, and thus acts to move a nucleus toward the ratio of neutrons to protons that has the least energy for a given total number of nucleons.
This consequently produces a more stable lower energy nucleus.
A Brief History of Radioactivity
A theoretical process of positron capture , analogous to electron capture, is possible in antimatter atoms, but has not been observed, as complex antimatter atoms beyond antihelium are not experimentally available. Shortly after the discovery of the neutron in , Enrico Fermi realized that certain rare beta-decay reactions immediately yield neutrons as a decay particle neutron emission. Isolated proton emission was eventually observed in some elements. It was also found that some heavy elements may undergo spontaneous fission into products that vary in composition.
In a phenomenon called cluster decay , specific combinations of neutrons and protons other than alpha particles helium nuclei were found to be spontaneously emitted from atoms. Other types of radioactive decay were found to emit previously-seen particles, but via different mechanisms. An example is internal conversion , which results in an initial electron emission, and then often further characteristic X-rays and Auger electrons emissions, although the internal conversion process involves neither beta nor gamma decay. A neutrino is not emitted, and none of the electron s and photon s emitted originate in the nucleus, even though the energy to emit all of them does originate there.
Internal conversion decay, like isomeric transition gamma decay and neutron emission, involves the release of energy by an excited nuclide, without the transmutation of one element into another. Rare events that involve a combination of two beta-decay type events happening simultaneously are known see below. Any decay process that does not violate the conservation of energy or momentum laws and perhaps other particle conservation laws is permitted to happen, although not all have been detected. An interesting example discussed in a final section, is bound state beta decay of rhenium In this process, beta electron-decay of the parent nuclide is not accompanied by beta electron emission, because the beta particle has been captured into the K-shell of the emitting atom.
An antineutrino is emitted, as in all negative beta decays. Radionuclides can undergo a number of different reactions. These are summarized in the following table. A nucleus with mass number A and atomic number Z is represented as A , Z. The column "Daughter nucleus" indicates the difference between the new nucleus and the original nucleus.
If energy circumstances are favorable, a given radionuclide may undergo many competing types of decay, with some atoms decaying by one route, and others decaying by another. An example is copper , which has 29 protons, and 35 neutrons, which decays with a half-life of about This isotope has one unpaired proton and one unpaired neutron, so either the proton or the neutron can decay to the opposite particle. The excited energy states resulting from these decays which fail to end in a ground energy state, also produce later internal conversion and gamma decay in almost 0.
More common in heavy nuclides is competition between alpha and beta decay. The daughter nuclides will then normally decay through beta or alpha, respectively, to end up in the same place. Radioactive decay results in a reduction of summed rest mass , once the released energy the disintegration energy has escaped in some way. Although decay energy is sometimes defined as associated with the difference between the mass of the parent nuclide products and the mass of the decay products, this is true only of rest mass measurements, where some energy has been removed from the product system.
The decay energy is initially released as the energy of emitted photons plus the kinetic energy of massive emitted particles that is, particles that have rest mass. If these particles come to thermal equilibrium with their surroundings and photons are absorbed, then the decay energy is transformed to thermal energy, which retains its mass. Decay energy therefore remains associated with a certain measure of mass of the decay system, called invariant mass , which does not change during the decay, even though the energy of decay is distributed among decay particles. The energy of photons, the kinetic energy of emitted particles, and, later, the thermal energy of the surrounding matter, all contribute to the invariant mass of the system.
Thus, while the sum of the rest masses of the particles is not conserved in radioactive decay, the system mass and system invariant mass and also the system total energy is conserved throughout any decay process. This is a restatement of the equivalent laws of conservation of energy and conservation of mass. Although these are constants, they are associated with the statistical behavior of populations of atoms. In consequence, predictions using these constants are less accurate for minuscule samples of atoms.
Radioactivity is one very frequently given example of exponential decay. The law describes the statistical behaviour of a large number of nuclides, rather than individual atoms. In the following formalism, the number of nuclides or the nuclide population N , is of course a discrete variable a natural number —but for any physical sample N is so large that it can be treated as a continuous variable.
Differential calculus is used to model the behaviour of nuclear decay. The mathematics of radioactive decay depend on a key assumption that a nucleus of a radionuclide has no "memory" or way of translating its history into its present behavior. A nucleus does not "age" with the passage of time. Thus, the probability of its breaking down does not increase with time, but stays constant no matter how long the nucleus has existed.
This constant probability may vary greatly between different types of nuclei, leading to the many different observed decay rates. However, whatever the probability is, it does not change. This is in marked contrast to complex objects which do show aging, such as automobiles and humans. These systems do have a chance of breakdown per unit of time, that increases from the moment they begin their existence.
The decay of an unstable nucleus is entirely random in time so it is impossible to predict when a particular atom will decay.
Catalog Record: A brief account of radio-activity | Hathi Trust Digital Library
However, it is equally likely to decay at any instant in time. The negative sign indicates that N decreases as time increases, as the decay events follow one after another. The solution to this first-order differential equation is the function:. The number of decays observed over a given interval obeys Poisson statistics. Now consider the case of a chain of two decays: The previous equation cannot be applied to the decay chain, but can be generalized as follows. Since A decays into B , then B decays into C , the activity of A adds to the total number of B nuclides in the present sample, before those B nuclides decay and reduce the number of nuclides leading to the later sample.
In other words, the number of second generation nuclei B increases as a result of the first generation nuclei decay of A , and decreases as a result of its own decay into the third generation nuclei C. The subscripts simply refer to the respective nuclides, i. Solving this equation for N B gives:. This case is perhaps the most useful, since it can derive both the one-decay equation above and the equation for multi-decay chains below more directly. For the general case of any number of consecutive decays in a decay chain, i.
D , each nuclide population can be found in terms of the previous population. Using the above result in a recursive form:. The general solution to the recursive problem is given by Bateman's equations: In all of the above examples, the initial nuclide decays into just one product. For example, in a sample of potassium , We have for all time t:. Differentiating with respect to time:. The above equations can also be written using quantities related to the number of nuclide particles N in a sample;.
In a radioactive decay process, this time constant is also the mean lifetime for decaying atoms. A more commonly used parameter is the half-life. Given a sample of a particular radionuclide, the half-life is the time taken for half the radionuclide's atoms to decay. For the case of one-decay nuclear reactions:. This relationship between the half-life and the decay constant shows that highly radioactive substances are quickly spent, while those that radiate weakly endure longer.
The factor of ln 2 in the above relations results from the fact that the concept of "half-life" is merely a way of selecting a different base other than the natural base e for the lifetime expression. The following equation can be shown to be valid:. They reflect a fundamental principle only in so much as they show that the same proportion of a given radioactive substance will decay, during any time-period that one chooses. A sample of 14 C has a half-life of 5, years and a decay rate of 14 disintegration per minute dpm per gram of natural carbon. If an artifact is found to have radioactivity of 4 dpm per gram of its present C, we can find the approximate age of the object using the above equation:.
The radioactive decay modes of electron capture and internal conversion are known to be slightly sensitive to chemical and environmental effects that change the electronic structure of the atom, which in turn affects the presence of 1s and 2s electrons that participate in the decay process.
Navigation
A small number of mostly light nuclides are affected. In 7 Be, a difference of 0. In , Jung et al. Rhenium is another spectacular example. A number of experiments have found that decay rates of other modes of artificial and naturally occurring radioisotopes are, to a high degree of precision, unaffected by external conditions such as temperature, pressure, the chemical environment, and electric, magnetic, or gravitational fields.
Recent results suggest the possibility that decay rates might have a weak dependence on environmental factors. It has been suggested that measurements of decay rates of silicon , manganese , and radium exhibit small seasonal variations of the order of 0.
- In this Section?
- Navigation menu.
- Brouhaha in Brooklyn: The Trail Of The Missing Corpses (Gotham Chronicles Book 2).
- The nature of radioactive emissions?
- Vermiculture Technology: Earthworms, Organic Wastes, and Environmental Management.
- A Brief History of Radioactivity | radioactive monticello!
An unexpected series of experimental results for the rate of decay of heavy highly charged radioactive ions circulating in a storage ring has provoked theoretical activity in an effort to find a convincing explanation. The rates of weak decay of two radioactive species with half lives of about 40 s and s are found to have a significant oscillatory modulation , with a period of about 7 s. As the decay process produces an electron neutrino , some of the proposed explanations for the observed rate oscillation invoke neutrino properties.
Initial ideas related to flavour oscillation met with skepticism. The neutrons and protons that constitute nuclei, as well as other particles that approach close enough to them, are governed by several interactions. The strong nuclear force , not observed at the familiar macroscopic scale, is the most powerful force over subatomic distances. The electrostatic force is almost always significant, and, in the case of beta decay , the weak nuclear force is also involved. The combined effects of these forces produces a number of different phenomena in which energy may be released by rearrangement of particles in the nucleus, or else the change of one type of particle into others.
These rearrangements and transformations may be hindered energetically, so that they do not occur immediately. In certain cases, random quantum vacuum fluctuations are theorized to promote relaxation to a lower energy state the "decay" in a phenomenon known as quantum tunneling. Radioactive decay half-life of nuclides has been measured over timescales of 55 orders of magnitude, from 2. The decay process, like all hindered energy transformations, may be analogized by a snowfield on a mountain.
While friction between the ice crystals may be supporting the snow's weight, the system is inherently unstable with regard to a state of lower potential energy. A disturbance would thus facilitate the path to a state of greater entropy ; the system will move towards the ground state, producing heat, and the total energy will be distributable over a larger number of quantum states thus resulting in an avalanche.
The total energy does not change in this process, but, because of the second law of thermodynamics , avalanches have only been observed in one direction and that is toward the " ground state " — the state with the largest number of ways in which the available energy could be distributed. Such a collapse a gamma-ray decay event requires a specific activation energy. For a snow avalanche, this energy comes as a disturbance from outside the system, although such disturbances can be arbitrarily small. In the case of an excited atomic nucleus decaying by gamma radiation in a spontaneous emission of electromagnetic radiation, the arbitrarily small disturbance comes from quantum vacuum fluctuations.
A radioactive nucleus or any excited system in quantum mechanics is unstable, and can, thus, spontaneously stabilize to a less-excited system. The resulting transformation alters the structure of the nucleus and results in the emission of either a photon or a high-velocity particle that has mass such as an electron, alpha particle , or other type.
According to the Big Bang theory , stable isotopes of the lightest five elements H , He , and traces of Li , Be , and B were produced very shortly after the emergence of the universe, in a process called Big Bang nucleosynthesis. These lightest stable nuclides including deuterium survive to today, but any radioactive isotopes of the light elements produced in the Big Bang such as tritium have long since decayed. Isotopes of elements heavier than boron were not produced at all in the Big Bang, and these first five elements do not have any long-lived radioisotopes.
Thus, all radioactive nuclei are, therefore, relatively young with respect to the birth of the universe, having formed later in various other types of nucleosynthesis in stars in particular, supernovae , and also during ongoing interactions between stable isotopes and energetic particles. For example, carbon , a radioactive nuclide with a half-life of only 5, years, is constantly produced in Earth's upper atmosphere due to interactions between cosmic rays and nitrogen. Nuclides that are produced by radioactive decay are called radiogenic nuclides , whether they themselves are stable or not.
There exist stable radiogenic nuclides that were formed from short-lived extinct radionuclides in the early solar system. Radioactive decay has been put to use in the technique of radioisotopic labeling , which is used to track the passage of a chemical substance through a complex system such as a living organism.
A sample of the substance is synthesized with a high concentration of unstable atoms. The presence of the substance in one or another part of the system is determined by detecting the locations of decay events. On the premise that radioactive decay is truly random rather than merely chaotic , it has been used in hardware random-number generators.
Because the process is not thought to vary significantly in mechanism over time, it is also a valuable tool in estimating the absolute ages of certain materials. For geological materials, the radioisotopes and some of their decay products become trapped when a rock solidifies, and can then later be used subject to many well-known qualifications to estimate the date of the solidification. These include checking the results of several simultaneous processes and their products against each other, within the same sample.
In a similar fashion, and also subject to qualification, the rate of formation of carbon in various eras, the date of formation of organic matter within a certain period related to the isotope's half-life may be estimated, because the carbon becomes trapped when the organic matter grows and incorporates the new carbon from the air. Thereafter, the amount of carbon in organic matter decreases according to decay processes that may also be independently cross-checked by other means such as checking the carbon in individual tree rings, for example.
The Szilard—Chalmers effect is defined as the breaking of a chemical bond between an atom and the molecule that the atom is part of, as a result of a nuclear reaction of the atom. The effect can be used to separate isotopes by chemical means. Radioactive primordial nuclides found in the Earth are residues from ancient supernova explosions that occurred before the formation of the solar system. They are the fraction of radionuclides that survived from that time, through the formation of the primordial solar nebula , through planet accretion , and up to the present time.
The naturally occurring short-lived radiogenic radionuclides found in today's rocks , are the daughters of those radioactive primordial nuclides. Another minor source of naturally occurring radioactive nuclides are cosmogenic nuclides , that are formed by cosmic ray bombardment of material in the Earth's atmosphere or crust. The decay of the radionuclides in rocks of the Earth's mantle and crust contribute significantly to Earth's internal heat budget. The daughter nuclide of a decay event may also be unstable radioactive.
In this case, it too will decay, producing radiation. The resulting second daughter nuclide may also be radioactive. This can lead to a sequence of several decay events called a decay chain see this article for specific details of important natural decay chains. Eventually, a stable nuclide is produced. Any decay daughters that are the result of an alpha decay will also result in helium atoms being created.
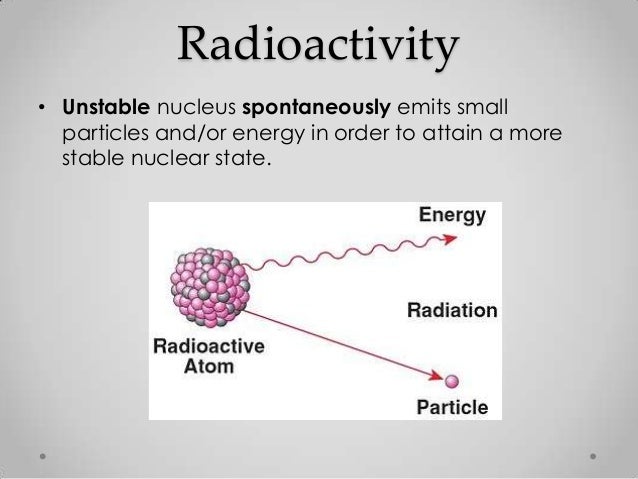
Some radionuclides may have several different paths of decay. Both thallium and polonium are radioactive daughter products of bismuth , and both decay directly to stable lead From Wikipedia, the free encyclopedia. For particle decay in a more general context, see Particle decay.